Lithium-ion (Li-ion) batteries have become the cornerstone of modern energy storage, powering everything from smartphones to electric vehicles. A critical component of these batteries is the cathode, where lithium ions move during discharge, and the choice of cathode materials directly influences the battery's performance, longevity, and safety. The cathode is derived from precursor materials, which are typically transition metal compounds. These precursors undergo several chemical processes to produce the active cathode materials, which then play a crucial role in the overall characteristics of the battery.
Chemical composition, crystalline quality, particle size and particle shape are the key parameters governing the quality and process efficiency of the cathode precursor materials.
Our solutions
Types of cathode precursors
- Nickel-Cobalt-Manganese (NCM) and Nickel-Cobalt-Aluminium (NCA) Precursors
NCM and NCA are among the most popular cathode materials in the industry, especially for electric vehicles. The precursors for these materials are mixed hydroxides, denoted as NixCoyMn(1-x-y)(OH)2 for NCM and NixCoyAl(1-x-y)(OH)2 for NCA. - Lithium Cobalt Oxide (LCO) Precursor
Lithium cobalt oxide (LiCoO2) is widely used in portable electronics. The precursor, cobalt oxide (Co3O4), is processed with lithium carbonate or lithium hydroxide to produce the final cathode material. - Lithium Iron Phosphate (LFP) Precursor
Lithium iron phosphate (LiFePO4) cathodes, used in EV batteries, are derived from iron phosphate (FePO4) precursors. - Lithium Manganese Oxide (LMO) Precursor
Manganese oxide (Mn3O4) serves as the precursor for lithium manganese oxide (LiMn2O4), a material known for its high-rate capability and good thermal stability, often used in hybrid vehicles and power tools. - Nickel-Rich Precursors
With the push for higher energy densities in batteries, nickel-rich precursors are gaining prominence. These precursors, often represented by Ni(OH)2 with varying amounts of dopants, are used to produce cathode materials with high nickel content.
Precursor synthesis and processing
The most common method for synthesizing cathode precursors is co-precipitation, where aqueous solutions of the metal salts are mixed to form liquid precursor. A precipitating agent, such as sodium hydroxide (NaOH) or ammonium hydroxide (NH4OH), is added to the solution, causing the metals to precipitate out as hydroxides.
Co-precipitation is a slow process – starting with nucleation, followed by the growth of primary particles, and finally agglomeration to larger secondary particles. The whole process can take anywhere between 20 to 40 hours, depending on process efficiency.
The temperature, pH, concentration and stirring speed of the solution are carefully controlled to ensure uniform particle size and composition of the precipitate. The precipitated product is typically a mixed metal hydroxide or carbonate, such as NixCoyMn(1-x-y)(OH)2 for NCM precursors. This precursor is then mixed with LiOH and calcined at 900oC to make the final cathode material. The process is schematically shown below.
Many parameters – including slurry composition, pH, temperature, and stirring speed – affect co-precipitation efficiency. Optimizing these parameters plays a key role in the quality and throughput of battery cathode precursor materials. To monitor and control them in real-time to improve the efficiency of the co-precipitation process, we offer a range of analytical solutions. What’s more, our solutions can also help you to ensure that your precursor material has the desired properties for further processing into the active cathode material.
How can I optimize my cathode precursor materials?
Cathode precursor quality and throughput can be optimized by measuring and controlling the following parameters:
- Particle size
- Particle shape
- Chemical composition and impurities
- Crystalline phase analysis
- Zeta potential
Find out more about each parameter below.
Particle size
Precursor particles nucleate, grow and then agglomerate to form larger secondary particles. To ensure the highest production efficiency, these particles should grow above their target size in the minimum possible time. The measurement of particle size over time is therefore an important way to fine-tune process parameters in slurry reactor.
Mastersizer range
The smartest way to measure particle size
Laser Diffraction is one of the best ways to accurately measure particle size distribution for process and quality control. Trusted by battery cell and battery material manufacturers worldwide, Mastersizer 3000 has become the de facto standard for particle size analysis.
The Mastersizer range of laser diffraction particle size analyzers set the benchmark for delivering rapid, accurate particle size distributions for both wet and dry dispersions. And it’s got even better with the introduction of the NEW Mastersizer 3000+ with advanced functionalities like Size Sure and Data Quality Guidance.
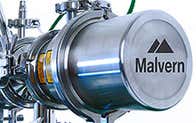
Insitec range
Robust, reliable, real-time particle sizing
Our online automated Insitec particle size analyzer is ideal for making these measurements in a production environment, delivering real-time analysis every few seconds.
Using a feedback loop, this information can be used to adjust parameters like Ph, temperature, or stirring speed.
What’s more, it can also ensure synergy with smart manufacturing process flows. This delivers high returns: typically, a cathode manufacturing plant producing 1000 kg of cathode material per day can save up to 200,000 USD per year by analyzing precursor slurry particle size with the Insitec.
Particle shape
Particle shape plays an important role in the formation of stable secondary particles and can significantly influence precursor yield (tap density), as well as the quality of the final cathode material. For example, elongated particles are more likely to break and re-dissolve in a slurry stirred at high speed.
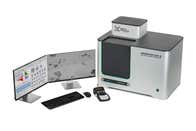
Morphologi 4
Rapid, automated particle size and particle shape analysis
To enable manufacturers to analyze and optimize particle shape, our Morphologi 4 optical imaging tool can be used to measure parameters such as circularity, elongation/aspect ratio, Circular Equivalent (CE) diameter, and transparency, with its fully automated image analysis.
Chemical composition and impurities
To achieve optimal chemical composition in the final cathode materials, it must first be controlled at the precursor level. X-ray fluorescence (XRF), which can analyze the chemical composition and impurities from just a few ppm all the way up to 100%, is the best technique for analyzing chemical composition.
XRF provides a simpler and more accurate way of measuring elemental composition than inductively coupled plasma (ICP) mass spectrometry, as it does not require any sample dilution or acid digestion. Many leading battery companies use our benchtop Epsilon 4 EDXRF or Zetium WDXRF spectrometers to analyze the composition of their precursor and cathode materials.
Complementing these is our new Revontium high-end EDXRF system. Combined with our set of battery reference standards and Forj / Egon 2 fusion systems along with our expertise, we offer a complete solution that can match or even surpass the accuracy and precision of ICP analysis.
Online elemental composition analysis solutions include Epsilon Xflow for battery liquid precursors and Epsilon Xline for analyzing the electrode coating.
Crystalline phase analysis of cathode precursor materials
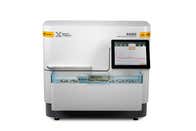
Aeris
The future is compact
Crystalline phase refers to the structure of materials at the atomic scale – the scale at which ionic or electronic transport happens or is hindered. The crystalline phase composition of the precursor can provide an early indication of the final cathode material’s quality.
To accurately analyze the crystalline phase composition of cathode precursor materials, manufacturers can use our Aeris compact X-ray diffractometer, an easy-to-use instrument with superb data quality.
Zeta potential analysis
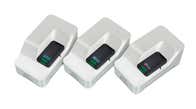
Zetasizer Advance Range
Light Scattering for every application
The precipitation of particles from the cathode precursor solution relies on primary particles (of 50-100 nm) interacting to form larger secondary particles (of 10-20 µm). Zeta potential can be used to analyze and adjust Ph and temperature values in order to optimize these interactions.
Our Zetasizer accurately measures zeta potential, and can also complement your R&D on precursor synthesis.
Further reading
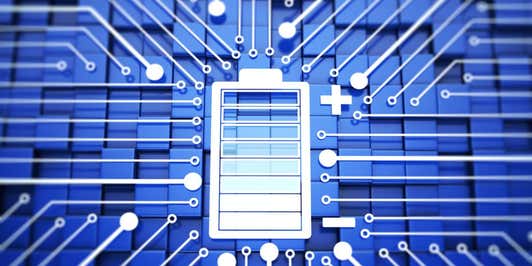
What XRD configuration shall one use to analyze battery cathode materials?
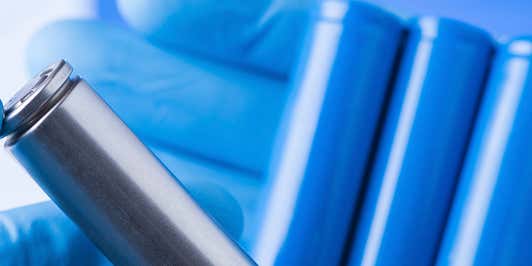
Analytical toolkit for the optimization of battery electrode materials
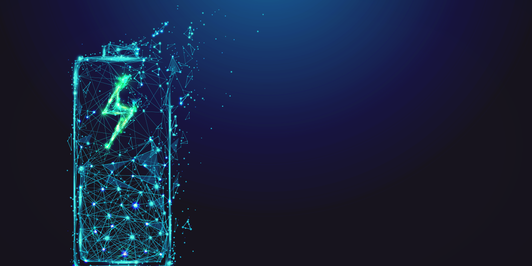
Battery manufacturing: Reliable, adaptable particle sizing using the Mastersizer 3000+
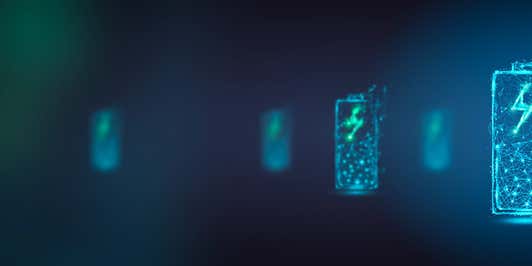
XRF Analysis of Lithium Iron Phosphate Cathodes
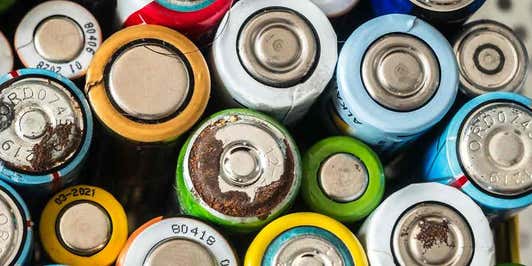
Lithium-ion battery recycling
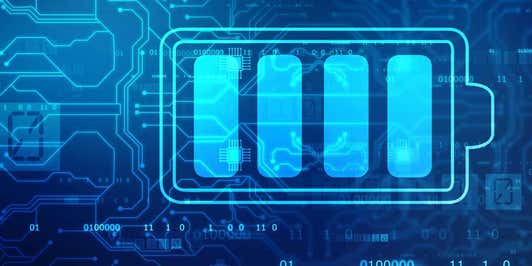